Photocatalysts can utilize sunlight to produce hydrogen from water splitting and to produce solar fuels through CO2 reduction, but the essential mechanism, how photogenerated charges are involved in the photocatalysis processes, has remained elusive since the beginning of photocatalysis study.
Although enormous efforts have been made in photocatalysts preparation and photocatalytic reaction tests in the past a half century of photocatalysis study, the mystery on the exact mechanism behind the process and how to make the process more effectively, do not actually know, as photocatalysts have complex nanoscale morphology features and particularly photogenerated charges in photocatalysis experiences broad lifetimes and pace, across femtosecond to seconds and subnano-meter to micro-meter.
Now, the mystery is resolved. Researchers in China have mapped the spatiotemporal evolution of charge transfers in cuprous oxide photocatalyst particles (a typical photocatalysts composed of only one copper atom and one oxygen atom), integrating three different techniques to reveal a holistic picture of the complex charge-transport mechanisms.
The team published their results on Oct. 12 in Nature.
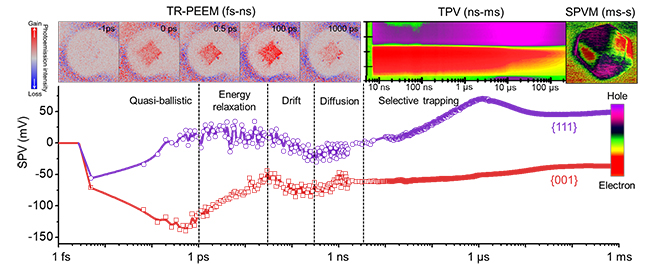
Photovoltage measurements on photocatalyst particles are used to spatiotemporally track the charge transfer processes on the femtosecond to second timescale at the single-particle level (Image by CHEN Ruotian)
"Water-splitting reactions with photocatalyst particles are a promising route for solar hydrogen, we have focused on the photogenerated charge dynamics in photocatalysis for decades," said co-corresponding author LI Can, a professor from the Dalian Institute of Chemical Physics (DICP) of the Chinese Academy of Sciences (CAS). "We believed that the photoinduced charges transfer and transport in photocatalysts, and from bulk to surface reaction sites is the key determining the photocatalytic efficiency; however, it is challenging to understand the mechanisms which spans a wide spatiotemporal range from subnanometers to micrometers and from femtoseconds to seconds."
According to LI, to thoroughly track this process in a photocatalyst particle has long been thought to be a dream, and the research team has finally achieved their goal.
"Photocatalysis generally starts with harvesting solar energy in the particulate semiconductor material, which transforms absorbed solar photons into excited charges (electrons and holes). These excited charges participate in the photocatalytic reactions," said co-corresponding author FAN Fengtao, a professor from DICP. "The photo-generated electrons and holes require separation from their initial states and transfer to a catalyst surface, where they initiate reactions to produce chemical fuels."
To transfer to the surface, the electron or hole are derived from photo-excitation, which triggers the separation from their ground states. According to Fan, the efficiency challenge arises because, at such tiny physical scales, the photocatalysts often lack the forces needed to separate the charges. Efficient separation requires a dependable electric field.
"To form an aligned electric field in the photocatalyst particle, we carefully engineered the morphology of cuprous oxide particles," said the first author, CHEN Ruotian Ph.D. from DICP.
Called facet engineering, the work consisted of precisely tuning the ratio between two of the facets on the particles, morphing them from cubes to octahedrons and generating built-in electric fields that can aid in charge separation.
"Interestingly, we found that the built-in electric fields vary with the facet ratios and become prominent in the configuration as the ratio increases," CHEN said, explaining that the observed inter-facet potential differences correlate with why the charge transfer can vary in magnitude in different directions. "This phenomenon, called anisotropic charge transfer, appears optimized in the truncated octahedral configuration. However, only electrons are visible on the surface after this facet tuning, indicating that holes hardly transfer to the surface and may limit efficiency."
The researchers introduced hydrogen into the photocatalyst particles, forming defects that offer a potential pathway for hole transfer to surface via a selective trapping process, which prevents the hole from recombining with an electron.
"We found that defects were selectively incorporated into the facets of the particles, thus allowing the hole transfer selectively to defected facets." FAN said. "Photovoltage measurements indicated that the selective defect incorporation further contributes to efficient charge separation. We also demonstrated that the photogenerated electrons and holes can be selectively extracted to specific facets, but the mechanism underlying this process is likely different for holes than for electrons."
To better understand the spatiotemporal origin of the efficient charge separation within the nanosecond range, the researchers used time-resolved photoemission electron microscopy —The resulting images verified that photoelectron density varies on different facets and that the electrons move from one facet to another on ultrafast time scales.
"However, ultrafast transport is not expected from the conventional drift-diffusion model, even though it has long been regarded as the dominant charge separation mechanism in photocatalysis," CHEN said, referring to the idea that a charge could drift around a particle, repeatedly scattering as it encounters another component. "We ascribed the ultrafast charge transfer to the ballistic transport regime, in which the carriers propagated at extremely high speeds, as the carriers travel the length of the particle prior to scattering."
Following the ultrafast charge transfer, the researchers observed a gradual increase in electrons on both facets, likely arising from the drift of electrons from bulk to the surface. To directly observe the charge transfer process, the researchers performed a transient photovoltage analysis and found that signals shifted from negative to positive as the timescale progressed from nano- to microseconds.
"We saw ultrafast ballistic electron transfer to one type of facet and slow defect-induced hole transfer to the other type of facet, so we can determine that the efficient spatial separation of photogenerated electrons and holes on the facets was due to the spatiotemporally anisotropic charge transfer mechanism," FAN said. "We demonstrated that the quasi-ballistic inter-facet electron transfer and spatially selective trapping are the dominant processes facilitating efficient charge separation in photocatalysis."
Taken together, according to CHEN, FAN and LI, this study suggests that the dominant photocatalytic mechanisms could be manifested and tuned through rational engineering of anisotropic facets and defects.
"We combined three different methods — time-resolved photoemission microscopy (femto- to nanoseconds), transient surface photovoltage spectroscopy (nano- to microseconds) and surface photovoltage microscopy (microseconds to seconds) — like a relay race, for the first time, to follow the entire mechanism of electron and hole to surface reaction centers in a single photocatalyst particle," LI said. "The ability to spatiotemporally track charge transfer enables advancing experimental technique for understanding the complex mechanisms in energy conversion devices, paving the way for the rational design of photocatalysts with improved performance."
CHEN Ruotian and REN Zefeng are the co-first authors. LI is also affiliated with the University of CAS and the University of Science and technology of China (USTC). Other cooperations include Shandong University and Helmholtz-Center Berlin for Materials and Energy.
The Fundamental Research Center of Artificial Photosynthesis, funded by the National Natural Science Foundation of China; CAS Projects for Young Scientists in Basic Research; National Program on Key Basic Research Project; and DICP Innovation Foundation supported this work.